Fab labs in education: Difference between revisions
m (→Bibliography) |
m (→Bibliography) |
||
Line 362: | Line 362: | ||
* Vilbrandt, T., Malone, E., Lipson H., Pasko, A., (2008) "Universal Desktop Fabrication", in Heterogeneous Objects Modeling and Applications, pp. 259-284. DOI: [http://dx.doi.org/10.1007/978-3-540-68443-5 10.1007/978-3-540-68443-5] {{ar}} | * Vilbrandt, T., Malone, E., Lipson H., Pasko, A., (2008) "Universal Desktop Fabrication", in Heterogeneous Objects Modeling and Applications, pp. 259-284. DOI: [http://dx.doi.org/10.1007/978-3-540-68443-5 10.1007/978-3-540-68443-5] {{ar}} | ||
* Walter-Herrmann J, Büching C, editors. ''FabLab: Of machines, makers and inventors''. transcript Verlag; 2014. | |||
* Wohlers, T. (2005). 3D Printing in Education: How High Schools, Colleges, and Universities Leverage 3D Printing Technology.â Time-Compression Technologies, Sept/Oct 2005. [http://wohlersassociates.com/SepOct05TCT3dp.htm HTML] | * Wohlers, T. (2005). 3D Printing in Education: How High Schools, Colleges, and Universities Leverage 3D Printing Technology.â Time-Compression Technologies, Sept/Oct 2005. [http://wohlersassociates.com/SepOct05TCT3dp.htm HTML] |
Revision as of 16:51, 23 January 2017
Introduction
This article attemps to summarize some of the literature related to fab labs, maker spaces and similar setups in education. A synthesis may (later) appear in Digital design and fabrication in education. This is a note taking piece - Daniel K. Schneider 18:58, 24 November 2011 (CET).
See also:
- Fab lab (Technical and political overview)
- Tour de Fablab (Some resources for demos/workshops)
- 3D printers in education
- laser cutting in education
- Computerized embroidery in education
- Design and technology in England's national curriculum
- Digital design and fabrication in education
Fab labs are usually outreach programs, i.e. addressing a population at large and not specifically schools. However, the fab lab movement seems to inspire schools and "private fab labs" are sprining up, or older existing structures are being "digitized". Such Fab labs in education seem to follow several connected axis of development. For example:
- Teaching technology and design for its own sake following a political and cultural agenda, i.e. aims can be summarized as empowerment. This is the thrust of original fab lab and rather concerns schools in the developing countries.
- Teaching of "design" through modeling and physical realization
- Creation of manipulables (e.g. bricks of a warehouse) or persona (for role playing). In this case the fab lab users can be both teachers and learners.
- Models for teaching about past or present artifacts or abstract concepts like chemistry models. Again this can concern just teachers or also learners.
- "Learning is making", a stance adopted in constructionism à la Papert
In addition, we should point out that fablab-like workshops in some school systems are not necessarily called fab labs. Fab labs are just one way of labeling and thinking about design and fabrication facilities.
Overview
Some history
Fabrication laboratories (fab labs) did exist for a long time as a facility attached to research and development units in larger organizations. Educational and public fab labs however, were created much later under the impulse of Neil Gershenfeld at the Massachusetts Institute of Technology (MIT). In the 1990s, he organized a course called How to Make (Almost) Anything within which students has access to a state-of-the-art fabrication lab. Gershenfeld and his collaborators later developed a world-wide outreach program for providing digital fabrication tools for rapid prototyping. According to Gordon (2011), “Gershenfeld thought Fab Labs would bring digital fabrication to everyone from hobbyists to inventors, entrepreneurs, displaced workers, small-business owners, and individuals in workforce-development programs. The goal: letting ordinary people turn an idea into a product for personal or commercial use by giving them access to design and manufacturing tools previously available only to engineers at large companies.”
Smaller initiatives sprung up in other universities, e.g. the Fab@Home project at Cornell University. Hod Lipson and Evan Malone built one of the first microwave-oven-sized 3D printers, which they called a “fabber.” Anyone can put a fabber together for a few hundred dollars and unlike the more popular RepRap models (see below) it can print several types of materials, including silicone, cement, stainless steel, cake frosting, and cheese.
Interestingly, other low-cost fabrication models have been developed in entirely different contexts. For example, the RepRap 3D printer is a fall-out from artificial intelligence research on auto-mimesis. Its pivotal role for digital design and fabrication in schools, homes and "hacker spaces" is described in the 3D printing article.
Fab labs grew out of academia. However, they reflect are more general technological and societal trend. As the power of desktop fabbers will increase and cost decrease over the next few years, Lipson et al. (2004:1032) argue that “more elaborate machines may be printable by a growing community around the world. Moreover, as new research leads to multimaterial functional freeform fabrication, we expect that incorporation of elastomers, lubricants, actuators, and sensors, electronics and power devices (Lipson, 2005b) will allow faithful replication and electronic sharing of an ever increasing scope of physical models and artifacts.”
This is “laying the foundation for an approach called “distributed mass manufacturing,” where individuals use personal-scale manufacturing machines at home to produce everything from simple objects like toothbrushes to complex parts such as machine components. It is said that the approach could help “democratize” technology, although it would probably augment, not replace, traditional manufacturing methods.” (Gordon, 2011).
While Fab labs are clearly a project that grew out of engineering and political engagement, other educational stake holders became interested, e.g. CAD/CAM teachers or educational technologists or just "ordinary" teachers trying to explore something different. Conceptual foundations for more articulated "fab lab pedagogies" can be rooted in various strands of constructionism or even older learning theories or educational models (e.g. Dewey and Freinet). But as we said before, fab labs do have a political and cultural agenda that is growing and becoming more popular in the last few years. The relative affordance of fabbing tools in the early 2000's gave birth to a round of new Research and Development (e.g. Andersen et al, 2005 or Gershenfeld, 2005). In 2011, prices for devices like a 3-D printer, an embroidery machine or a laser cutter, a low enough for school budgets or even individuals.
“Currently, there are Fab Labs in more than 22 countries around the world, ranging from Afghanistan, England, India, Kenya, Norway, South Africa, and Spain to Elyria, Ohio.” (Gordon, 2011).
The original M.I.T fab lab does have an impressive list of machinery. The "official" core capability of a fab lab should according to the (outdated) Fab LAB FAQ (retrieved 13:17, 25 November 2011 (CET)) includes:
- A computer-controlled laser cutter, for press-fit assembly of 3D structures from 2D parts
- A larger (4'x8') numerically-controlled milling machine, for making furniture- (and house-) sized parts
- A sign cutter, to produce printing masks, flexible circuits, and antennas
- A precision (micron resolution) milling machine to make three-dimensional molds and surface-mount circuit boards
- Programming tools for low-cost high-speed embedded processors
MIT's Fab Lab 2.0 hardware specification (retrieved 13:17, 25 November 2011 (CET)) identify ~$50k equipment and ~$10k materials, including:
- Mini 24" x 12" 35W laser cutter (epilog laser)
- Shop Bot, i.e. some kind of soft materials cutter/mill (Shopbot tools)
- 3D Printer (Up!)
- Milling Machine (Roland)
- A lot of smaller tools and also parts
The FABWiki yet includes another list.
Many fab labs have much less than that as you could see by looking at Labs List in the FabWiki. While the "official" web site put a lot of emphasis on subtractive manufacturing, small scale initiatives rather got for additive machining such as 3D printing or embroidery. This is normal since the latter are cheaper, easier to learn, designed to be made by skilled hobbyists (or sold by small low-tech start-ups) and not dangerous.
Anyhow, it is difficult to predict trends and even the current state of the situation without doing some serious field research. In addition, it seems that even at the "original institution", M.I.T. views change and other initiatives emerge. The website Machines that make and that also is sponsored by the MIT Center for Bits and Atoms hosting the fab lab project identifies a series of very low cost machines that either can make themselves, other machines, functional parts or fun stuff. These machines are currently (2011) being designed by students and may very well lead to a new set of personal fabricators that are low cost, easy to produce and to assemble, i.e. like 3D printers, but more general purpose. An interesting example design is the FAB in a box project.
Digital design is the other pole where interesting developments happen. In the not so distant past, many designers relied on either commercial software or re-purposed software made for other purposes (e.g. free 3D modelers made for designing virtual environments for solid models). The only exception were programs like Skeinforge needed to produce machine code from models.
As of 2011, much more commercial and open source software for non-industry users are available. E.g. MIT's Kokompe kit (curently in early development stage) aims to provide a complete tool chain for 3D design and control. Another, very different example is Autodesk 123, computer-aided design and manufacturing (CAD) software designed for end users (as of nov 2011, free for Beta testing).
Fab labs today may be a vector for many purposes including an attempts (among others) to raise interest for science and/or engineering education. In particular in the UK, many upper secondary schools are now being equipped with "light-weight" design and fabrication labs. The 1999 National Curriculum for England for the design and technology track sets out specific requirements.
One probably should not label these labs as "fab labs" since the intent is slightly different and their organizational setup is very different. Fab labs are essentially outreach programs, i.e. address the public at large and are mostly staffed by volunteers or temporarily paid graduate students. In the UK, qualified CAD/CAM teachers teach an official "design and technology" curriculum in lower secondary school. The rationale for making this a mandatory subject is economic.
The The Design and Technology association's Response to the national curriculum Review, retrieved 20:51, 25 November 2011 (CET) points out that we found missing very much from the official curriculum for 11-14 year olds, i.e. design and technology teaching as one of the means to re-industrialize a badly performing economy: “George Osborne concluded his March 2011 budget speech by stressing the importance of building a creative and manufacturing-based economy able to compete on the world stage, “We are only going to raise the living standards of families if we have an economy that can compete in the modern age. So this is our plan for growth. We want the words: „Made in Britain‟ „Created in Britain‟ „Designed in Britain‟ „Invented in Britain‟ to drive our nation forward. A Britain carried aloft by the march of the makers. That is how we will create jobs and support families.”, and “Design and technology is the only school subject in key stages 1-3 that gives young people an authentic taste of many of the creative and technical careers, and the associated practical skills.”
Read more in Design and technology in England's national curriculum
Raising interest for science education
Fab labs probably are better suited for teaching design (including art) and technology (including computer science and electronics). However, some subjects relate to technology that does require mastery of some high-school science subjects, e.g. basic physics.
Bringing emotion and motivation into activity designs
Often, the informal fab literature if quite full arguments stating that participants have "fun", are "motivated" etc. We didn't find any longterm research that analyzes these components in an educational setting. However, several pilot studies do exist.
Spendlove (2007) published a conceptual framework for looking at fab lab activities. He argues that the “broad aspirational aims of the subject, as outlined in the English national curriculum statement of importance for Design and Technology, can be achieved through recognising the powerful overarching concept of emotion within three emerging domains: Person, Process and Product.”. In other words, the authors identify three "emotional" domains within digital design and fabrication that should be addressed by educational design:
- E1 - the person domain: “there has to be sufficient recognition of the emotional underpinning required within the learner, person stage, which encourages uncertainty and nurtures risk taking within a divergent and creative environment.”
- E2 - the process domain: “for there to be true, meaningful and effective learning, there has to be sufficient recognition of the emotional investment required from the learner. This can only be achieved through discerning, significant and emotionally challenging and engaging contexts being facilitated.”
- E3 - the product domain: “there has to be emotional consideration for both the outcomes that evolve from the person and process domains in addition to considering the emotional needs of the receiver of such outcomes.”
These emotional components were then mapped to the British Design and Technology (QCA, 1999) statement of importance.
- Design and technology prepares pupils to participate in tomorrow’s rapidly changing technologies (E1, E2, E3)
- They learn to think and intervene creatively to improve quality of life (E1, E2, E3)
- The subject calls for pupils to become autonomous and creative problem solvers, as individuals and members of a team (E1, E2)
- They must look for needs, wants and opportunities and respond to them by developing a range of ideas and making products and systems (E2, E3)
- They combine practical skills with an understanding of aesthetics, social and environmental issues, function and industrial practices. As they do so, they reflect on and evaluate present and past design and technology, its uses and effects (E2, E3)
- Through design and technology, all pupils can become discriminating and informed users of products, and become innovators (E1, E3)
In order to avoid misunderstandings, we should make clear that this mapping just examines various aims that the British government defined for the mandatory design and technology programme for secondary schools can. It then suggests that each item should examined in terms of three emotional "domains" and then (of course) lead to appropriate educational "emotionally sound" designs.
Digital design is not emotional per se, but it does seem to have a high potential for raising emotions that in turn affect motivation.
In an interesting pilot study by Jonathan Fullwood (2002) based on sample of 23 schools, teachers believe that Pupils enjoy using CAD/CAM' (mean of 2.3 on a scale of 1-5, 1=highest).
Political, social, cultural issues
We already addressed the issue of raising interest for science engineering in primary and secondary school. (actually we didn't yet ..)
In this section we will address two specific issues that are strongly present in the fab lab literature: Opportunities of fab labs for developing countries and gender issues.
Development
According to Beyers and Koorbanally (2009), “failure to address the development of e-skills within the education system as well as the broader society will leave individuals on the wrong side of the digital divide as they cannot compete in a global economy”. This principles can be both applied to nations a whole but also to specific population groups in any country. The authors, using South Africa as an example, point out that it is no longer enough to "do well enough". “Using the South African example, in the 1960s South Africa represented 6% of the world's GDP. Today that figure is less than 0,5%, but year-on-year South Africa’s economy has never shrunk. South Africa has simply been out-innovated by other nations who had seen the signs, identified the trends, and acted accordingly. Today half of America’s economic growth comes from products that barely existed a decade ago! The degree to which this happens has become a key measure of national success. Innovation has become the economic religion of the 21st Century, but it’s no longer enough to differentiate one from ‘the bunch’. Radical innovation is a necessity to thrive today.”. Schools can't keep up, since the society formulates desirable sets of knowledge, skills, attitudes and values that will be outpaced by the society once a learner did finish school.
In order to define what is needed, the authors differentiate the vague "digital divide" concept into a double technological ladder that we present in table form below:
Level | School and business focus | Technology in society |
---|---|---|
4 | ICT enabled innovator | Technology enabled innovator |
3 | ICT practitioner | technology practitioner |
2 | ICT user (applications) | technology user |
1 | Basic literacy | technology literacy |
In this ladder, digital divide only concerns level 1, i.e. skills that most kids somewhat do acquire in most western countries and that won't make any real difference in competitivity. “Once basic literacy has been achieved, users need to be exposed to a variety of packages in order to become competent users. Beyond that is the mastery of the tools where ICT constitutes the main part of their future professions. The ultimate goal is the utilization of the power of ICTs to conceptualize and realize their creative talents in the form of innovations (and ultimately patents).” (Beyers and Koorbanally, 2009: 3). The authors also point out, that unfortunately, “computer literacy is largely confined to the development of psychomotor skills to operate macros of office packages and the transcription of information.” and that being computer literature does not necessarily translate into being e-literate which is beign defined as being able to create new knowledge from old. The Young Engineers and Scientists of Africa (YESA) initiatives that included a FabKids module for developing high productivity skills in a high-tech rapid-prototyping environment of a Fab Lab. Initial outcomes (include the fab lab, but also other initiatives) indicate that “learners, no matter what their background, are able to draw benefit from and operate in high-tech environments” (p. 8) and can use technology as a tool to solve challenges other than information retrieval.
Beyers (2010), identifies the pedagogy behind the South African FabKids project as rooted in Piaget, Dewey and Vygotsky. The authors argue that National Critical Outcomes “are met within this single project although initial FabKids encounters were of a limited nature due to time constraints of a single session”.
These South African National Curriculum statements include the typical higher-order cognitive skills that one can find in most curricla and that remain underdeveloped:
- identify and solve problems and make decisions using critical and creative thinking;
- work effectively with others as members of a team, group, organization and community;
- organize and manage themselves and their activities responsibly and effectively;
- collect, analyze, organize and critically evaluate information;
- communicate effectively using visual, symbolic and/or language skills in various modes;
- use science and technology effectively and critically showing responsibility towards the environment and the health of others; and
- demonstrate an understanding of the world as a set of related systems by recognizing that problem solving contexts do not exist in isolation
We don't believe that any educational system come close to implementing such goals. However, it can be argued that fab lab activities do engage students in tasks that do require several technical, meta-cognitive and social skills and therefore will to develop these. Beyers (2010:abstract) clearly emphases these multiple issues: “This [FabKids] project must be viewed in the context of a global shortage of key skills placing a higher priority on the initiation and development of a pipeline to attract youth into science, engineering and technology careers. Creativity and innovation feature high on the skills agenda but more importantly preliminary results indicate that learners from a broad range of schools were able to operate effectively in this post constructivist environment. Participants had to apply their knowledge, skill, attitudes and values in order to produce a solution to the challenges provided. The fundamentals of the design process of investigate, design, make, evaluate and communicate were emphasized where the FabKids had to draw on their own collective knowledge using a range of technologies available to them.”
Gender issues
Dledlo and Beyers (2009) describe an effort to address the issue of inequality in girls’ and women’s access to science, engineering and technology (SET) education and careers through raising awareness on SET among secondary school girls in South Africa. Other studies cited, e.g. Gilley and Begolly (2005), Haataja, Leinonenn and Tervonen (2006) or Graham and Smith (2005), identify a global issue, although the percentage of women engineers and scientiest is much higher in some countries than in others. Results from this study was qualitative and encouraged further development and follow-up studies. “The girls indicated that this was an interesting session that stimulated their interest in computers and technology. They indicated that they were keen to learn more about computers as a result of this stimulating exposure. They were excited by the sense of achievement in their ability to design a product from scratch and produce a high-quality prototype in such a short period of time. This brought them a degree of satisfaction” (Dledlo & Beyers, 2009:429). In addition this report discuss two other interesting issues: computer anxiety and participation anxiety. Fab lab participation seems to require “team work, research skills, communication skills, design skills, technical drawing skills, entrepreneurial skills, computer skills, creativity, innovation, thinking out of the box and cooperative learning”. Although, the study doesn't make the claim, we believe that these are stumbling blocks and need to be overcome by longer exposure in order to become opportunities.
Haataja et al (2006:36), in their recommendations report address the question how girls are different from boys. They seem to imply that girls-specific classes may be beneficial. “When allowed to acquaint themselves with non-traditional sectors within their own group, girls’ perceptions of their own competences improved. It was more common for them to select the advanced syllabus in mathematics or apply to a university of technology than among their peers. It was highly noticeable in girls-only groups that girls helped and encouraged each other, allowed each other the peace to concentrate and had an active approach to technology.”. The authors also point out that girls may have typical topical interests. “It is however, important to notice that 1) although the content, examples and practices must include topics and themes that interest girls, one must avoid stereotypes (e.g. not all girls are interested in fashion)” (ibid, p. 35). Or even more clearly, the reports ends with the following final words on "Why do woment need ICTs":
Women need ICTs for the same reasons as men: to get more information to carry out their productive, reproductive, and community roles; to conduct their businesses, as a service of employment and to work in the ICT industry; to find resources for themselves, their families, their work, and their communities; and to have a voice in their lives, their community, their government, and the larger world that shares their issues and problems. In summary, they need ICTs to function in a digital world.” – or as we say in WomanIT: to prevent technology bypassing women.
- Marja-Leena Haataja, Eija Leinonen, Marjo Riitta Tervonen (2006)Teaching of design and fabrication
As we mentioned before, fab labs were born in higher education (in particular Gershenfeld:2005) and most of these are sponsored by academic institutions. On the hand, in many countries, schools did offer facilities and even classes for all sorts of bricolage (e.g. wood working, metal working, knitting, cooking, etc.).
The impact of fab labs on the school system seems to be mostly cultural, i.e. design and fabrication is a "hip activity". There also is a technology impact, e.g. people who monitor technology like the author of article see the technology used in fablabs and then start thinking about using it in schools. Also, The most prominent low-cost fabber projects were founded by academics. E.g. RepRap by Adrien Bowyer et al. at University of Bath (UK); Fab@Home by Evan Malone while he was a PhD student at Cornell.
Since the new Design and technology in England's national curriculum, English schools can acquiring all sorts of hardware, e.g. laser cutters, hobby mills, embroidery and sewing machines, small 3D printers, etc.
Commercial low-cost printers are now being sold to design schools for their design classes. E.g. a Desktop Factory printer is advertised as “With the Desktop Factory 3D printer, departments within large firms will be able to have their own dedicated 3D printers, and many small businesses, design firms and schools will be able to own this capability for the first time. Professional designers, engineers and students alike will be able to build inexpensive models from their designs before committing to expensive, custom prototyping” (retrieved 17:30, 25 June 2009 (UTC)). Such printers are still too expensive for ordinary schools (about CHF/USD 15'000 and high material costs).
While we will not discuss in detail obvious application areas of "fab lab techniques" in research and design at the university level, there exist a number of areas where cheap "fab lab" technology and in particular 3-D printers could be successfully used. E.g. Allard (2006) argues that “3D printing has the potential to overcome the barriers to the widespread use of RP in biological anthropology. The 3DP technology is easy to use, fast and economical to operate making it well suited to supporting projects in biological anthropology. The main limitation of the use of 3DP is the level of awareness of how it can be used to enhance or facilitate projects in biological anthropology.”
Burry (2006) analysis Antoni Gaudi's approach to thinking, modeling, and making. “[...] in considering the model as a design instrument, and modelling as a design process, Gaudi has bequeathed us two bases for reflecting on how we make and use models today. The first is his creation of a unique process based on a geometrical codex whose value is shared by designer and builder alike. The second bequest is a process involving an apprenticeship that has stood the test of time inasmuch as the exact methods that he developed in his time are still in abundant use on the project today.”
These examples illustrate that there is interest for "rapid prototyping" techniques in diverse areas outside of engineering that maybe should be taught in higher secondary and universities, i.e. 3D scanning, modelling and 3D printing may enter curricula.
Models for education
Knapp et al. (2007) stress the benefits of physical models in a variety of educational settings, e.g. Mathematics, anatomy, molecular biology, aeronautics, chemistry, archeology: “Physical models have been shown to enhance learning in general student populations as well. Students learn in a variety of ways, and models allow students to include their sense of touch in the learning experience. The role of experience is emphasized in Piaget's description of cognitive development, that is, to know an object a subject must act on it and thus transform it - displace, connect, combine, take apart, and reassemble it. (Cohen, 1983).
Historic artifacts
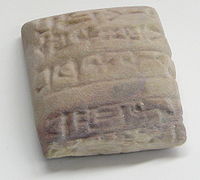
Lipson et al. (2004:1032) reports on “the use of computer-aided modeling tools and rapid prototyping technology to document, preserve, and reproduce in three dimensions, historic machines, and mechanisms. We have reproduced several preassembled, fully functional historic mechanisms such as early straight line mechanisms, ratchets, pumps, clock escapements and counting devices, including various kinematic components such as links, joints, gears, worms, nuts, bolts, and springs.”. The authors also argue that “One can realize many historical concepts that exist only on paper, such as Leonardo da Vinci's slider crank mechanism, as well as other models that exhibit more contemporary concepts from aerodynamics to molecular biology.”
Physical models of abstract concepts
Science education has a long tradition of using of models. If one goal of science education is to enhance and maximize an individual's special conceptual ability, then access to manipulatives is advisable for those individuals. This access to manipulatives might also enhance development of their logical abilities... Internality is positively correlated with student achievement, and experience with manipulatives tends to move external subjects toward the internal end of the internal external continuum. (Cohen, 1982)”. Knapp et al. also stress the general benefits of models and manipulatives, quoting a study from Lillard (2006) showing that children from a Montessori kindergarten significantly outperformed their peers at traditional schools in standardized tests of reading and math.
In mathematics education, for example, Eisenberg et al. already in 2005 made the following statement. “One of the most important shifts in mathematical crafts is due to the increased power and affordability of fabrication devices - essentially, new sorts of output devices - that work in conjunction with computers. (Cf. the recently-published book by Gershenfeld for an enthusiastic discussion.) There are a number of devices that are relevant to this theme, among which are: (i) laser cutters (which employ a laser to cut flat sheets of wood or plastic into desired shapes), (ii) 3D printers (which output 3-dimensional forms in plastic or plaster, among other possible materials), and (iii) computer-controlled sewing machines (which can embroider fabric according to computational control)”.
Among other benefits these authors point that “One usually-unheralded aspect of mathematical crafts (particularly in comparison to purely "virtual", computer-based activities) is that the use of tangible materials permits children to create objects and artifacts that populate their physical space. In classrooms, it is not all that unusual to see mathematical models placed on shelves, hung from the ceiling, assembled into mobiles, and so forth. The contrast with computer-based activities here is telling: a mathematical game or simulation may be marvelous, but it remains (for the most part) "hidden" inside the computer; unlike the physical products of crafts, which are simply present and continuous in children's spaces, an educational computer program is invisible unless consciously accessed.”
Building blocks for games and simulations
Computer supported physical manipulatives
A good example is the TinkerTable integrated learning environment. It is a “tabletop learning environment which allows apprentices to build small-scale models of a warehouse using physical objects like wooden shelves, docks and rooms as well as metallic pillars, all scaled at 1:16. The system is made of a 2m by 1.5m table covered with whiteboard material and a gallows carrying a camera, a projector and a mirror. The purpose of the camera is to track the position of objects on the table and transfer this information to a computer running a logistics simulation. The position of the object is obtained thanks to fiducial markers (similar to 2D barcodes) detected by StudierStube tracker. The projector is used to project information on the table and on top of the objects, indicating for example the accessibility of the content of each shelf or security zones around obstacles.” ( retrieved 13:44, 25 October 2009 (UTC))
“The TinkerLamp environment is a lighter and portable version of the TinkerTable. It consists of a projector and camera mounted in a metal casing which is suspended above a regular classroom table by an aluminum gooseneck. Shelves, pillars and docks are scaled at 1:48. The functionality of this small version is identical to the large version except for the size of the warehouse (32m by 24m on the TinkerTable but limited to 24m by 18m) on the TinkerLamp version”. The shelves (i.e. the manipulatives) were made with ABS plastic and could have been printed with a 3D printer.
Objects for the visually impaired
The Tactilelearning.org stresses the importance of tactile models for the visually impaired and present several devices made "by hand" for teaching. A similar project is Touch Graphics that produced a number of projects, some of which are commercially available.
Eisenberg et al (2005) demonstrated for example embedded computers within construction pieces that are used to create two dimensional and three-dimensional "cellular automaton kits". “By placing computers inside craft objects themselves, we can make those objects programmable, and can endow them with qualities of interactivity and autonomous behavior. In turn, this enables new sorts of mathematical content to be brought into the sphere of craft activities.”
Sharing
Several repositories now exist that allow people to share (and take). Most 3D printers rely on the STL format described above and that decomposes a 3D structure into slices. There exist several options to create such a printable 3D representation.
Since most (active) teachers do want to adapt designs to their own needs, sharing of the model is not enough. Source code also must be shared, and ideally designers in various sub-fields also should share the same tools and ways of producing artifacts. For example, models for math teaching can be created with the following workflow desribed by Knapp et al. (2008):
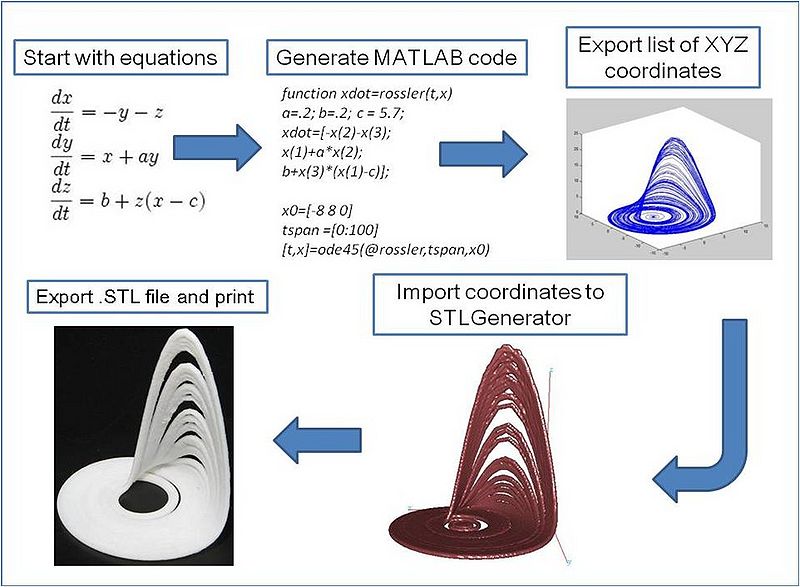
Others may use 3D modeling software or 3D scanners.
Links
- Brian Smith's website (includes a blog). The site includes a few examples of young children at making.
- Maker Education Initiative (Sponsored by Make Magazine)
- NuVu, Magnet Innovation Center for Young Minds. Includes projects centred around a "Design studio model".
- FabLab@School | Transformative Learning Technologies Lab (Paulo Blikstein, Stanford)
- Key Qualities for a School Makerspace by Parker Thomas, MAKE, Posted 2013/08/11
- dschool, Hasso Plattner, Institute of Design at Stanford (home page with various stories, resources, etc.)
- Make the Future Workshop Toolkit (pdf), made by WAAG for UNDP
- How the Maker Movement Is Moving Into Classrooms, EduTopia, July 2014
- The DIY World of Maker Tools and Their Uses, by Vicki Davis, Edutopia, July 2014
Bibliography
- Allard, Travis, T. (2006). The Role of 3D Printing in Biological Anthropology, University of Manitoba, Master Thesis, Allard 2006.pdf PDF
- Anderson-Rowland, M.R. (2003). Why aren’t there more women in engineering: can we really do anything, ASEE Annual conference proceedings, pp. 7295-7307.
- Andersen, D; C. Bennett, P. Huynh, L. Rassbach, S. Reardon, and M. Eisenberg (2005). Printing Out Trees: Toward the Design of Tangible Objects for Education, Proceedings of Education and Technology. PDF.
- Bereza, Marek (2007). Rise of the Replicator: The Evolution of Media Into The Tangible. The Royal College of Art, Master thesis. PDF
- Beyers, RN, Koorbanally, NA (2009). Computer literacy: insufficient for digital age literacy learners, eSkills Summit 2010, Cape Town, 26-28 July 2010, pp 10, http://hdl.handle.net/10204/4753
- Beyers, R.N., Creating a Sputnik Moment for Learners in Africa, in 2nd South African International Aerospace Symposium. 2008: Cape Town.
- Beyers, Ronald N. (2010). Nurturing Creativity and Innovation Through FabKids: A Case Study,Journal of Science Education and Technology, Volume 19, Number 5, 447-455, DOI: 10.1007/s10956-010-9212-0
- Breen, Jack; Robert Nottrot, Martijn Stellingwerff (2003). Tangible virtuality--perceptions of computer-aided and physical modelling, Automation in Construction, Volume 12, Issue 6, Design e-ducation: Connecting the Real and the Virtual, November 2003, Pages 649-653, ISSN 0926-5805, DOI: 10.1016/S0926-5805(03)00053-0.
- Burns M., (1995) The Freedom to Create, in Technology Management, Volume 1, Number 4 http://www.ennex.com/~fabbers/publish/199407-MB-FreedomCreate.asp
- Bowyer, Adrian (2007) Why Accountants are Dull and Guitarists are Glamourous - The End of Intellectual Property, Time Compression Technology Magazine, volume 15, issue 3, p33 (2007).
- Bowyer, Adrian (2007)- The Self-replicating Rapid Prototyper ─ Manufacturing for the Masses, Invited Keynote Address, Proc. 8th National Conference on Rapid Design, Prototyping & Manufacturing, Centre for Rapid Design and Manufacture, High Wycombe, June 2007. Rapid Prototyping and Manufacturing Association, ISBN-13: 978-0948314537 (2007).
- Bowyer, Adrian (2007). Breed your own Design, Icon Magazine, volume 52, October 2007.
- Burry, Mark; Michael Ostwald, Peter Downton, Andrea Mina, (2007) Homo Faber - Modelling Architecture, Sydeney: Archadia Press, ISBN 9780977571123. PDF (See also the video: Thinking modeling making
- Ceccarelli, M., (Ed.) 2000, International Symposium on History of Machines and Mechanisms, Proc. HMM Kluwer Academic, Dordrecht.
- Cohen D. L., Malone E., Lipson H., Bonassar L., (2006) "3D direct printing of heterogeneous tissue implants", Tissue Engineering, Vol. 12, No. 5: 1325-1335
- Cohen, H.G. (1983). A comparison of the affect of two types of student behavior with manipulatives on the development of projective spatial structures. Journal of Research in Science Teaching, 20(9), 875-883
- Cohen, H.G. (1982). Relationship between locus of control and the development of spatial conceptual abilities. Science Education, 66(4), 635-642
- Dlodlo, Nomusa and Ronald Noel Beyers (2009). The Experiences of South-African High-School Girls in a Fab Lab Environment, Proceedings Of World Academy Of Science, Engineering And Technology Volume 37 January 2009 Issn 2070-3740. PDF Reprint, CSIR Researchspace Abstract/PDF
- Editors' Review (2005). Desktop Factories - FAB The Coming Revolution on Your Desktop -- from Personal Computers to Personal Fabrication By Neil Gershenfeld, Basic Books, Business Week, May 2 2005.
- Eisenberg, Michael, Ann Eisenberg, Glenn Blauvelt, Susan Hendrix, Leah Buechley, and Nwanua Elumeze (2005). Mathematical Crafts for Children: Beyond Scissors and Glue, Proceedings of Art+Math=X Conference, Boulder, CO, pp. 61-65, 2005. Reprint: Department of Computer Science, University of Colorado. PDF
- Eisenberg, M. and Buechley, L. (2008). Pervasive Fabrication: Making Construction Ubiquitous in Education Journal of Software, 3:4, pp. 62-68. PDF
- Fullwook, Jonathan, CAD/CAM in Schools, The Journal of Design and Technology Education, 7 (1)
- Gershenfeld N. Think Globally, fabricate locally, PrincipalVoices.com. PDF (reprint)
- Gershenfeld, Neil, A., (2005) FAB: The Coming Revolution on Your Desktop – From Personal Computers to Personal Fabrication, Basic Books, ISBN 0-465-02745-8.
- Gilley, J., Begolly, J., (2005). Great progress, great divide: The need for evolution of the recruitment model for women in engineering, ASEE Annual conference and expose, pp.7003-7013.$
- Glenn, Joshua and Elisabeth Foy Larsen (2013). Unbored: The Power of 'Making' in the Classroom, Huffington Post, Education, HTML, retrieved Jan 6 2013.
- Gordon, Leslie, Rapid prototyping for the masses, Machine Design.com June 9, 2011 , retrieved 13:17, 25 November 2011 (CET) from http://insidepenton.com/machinedesign/June9RapidPrototypingArticle.pdf
- Graham, J.W., Smith, S.A. (2005). Gender differences in employment and earnings in science and engineering in the US, Economics of education review, pp. 341-354.
- Haataja, M., Leinonenn, E., Tervonen, M.R. (2005). University of Oulu/Kajaani University Consortium WomenIT team Finland. Exploring mechanisms to stimulate increased participation in science, engineering and technology: international experiences. PDF at http://women-in-ict.meraka.csir.co.za -
- Institute of the Future (2009). The future of making, PDF
- Kuznetsov, Stacey and Eric Paulos (2010). Rise of the Expert Amateur: DIY Projects, Communities, and Cultures, Proceedings of ACM NordiCHI 2010: Extending boundaris, Reykjavik, PDF preprint
- Jenweill, Mark, Fab Labs unshackle imaginations, USA Today, 11/6/2005.
- Jensen, Daniel; Chris Randell, John Feland, Martin Bowe (2002). A Study Of Rapid Prototyping For Use In Undergraduate Design Education, Proceedings of the 2002 American Society for Engineering Education Annual Conference & Exposition. PDF
- Knapp M., Wolff R., Lipson H. (2008), "Developing printable content: A repository for printable teaching models", Proceedings of the 19th Annual Solid Freeform Fabrication Symposium, Austin TX, Aug 2008. PDF.
- Lassiter S (2009) Vision on the road to the future. http://isites.harvard.edu/fs/docs/icb.topic558015.files/Lassiter-Vision-On%20the%20road%20to%20the%20future.pdf
- Lillard, A. and Else-Quest, N. (2006) The Early Years: Evaluating Montessori Education Science, Vol. 313. no. 5795, pp. 1893 - 1894
- Lipson H., Moon F.C., Hai J., Paventi C., (2004) 3D-Printing the History of Mechanisms, ASME Journal of Mechanical Design, pp. 1029-1033. PDF
- Lipson H. (2005a) "Homemade: The future of Functional Rapid Prototyping", IEEE Spectrum, feature article, May 2005, pp. 24-31 http://www.mae.cornell.edu/ccsl/papers/Spectrum05_Lipson.pdf
- Lipson H. (2005b). "Homemade: The future of Functional Rapid Prototyping", IEEE Spectrum, feature article, May 2005, pp. 24-31.
- Lipson (2007), Printed 3D Models for Customized Hands-On Education. Proceedings of Mass Customization and Personalization (MCPC) 2007.
- Lobovsky M., Lobovsky A., Behi M., Lipson H. (2008), "Solid Freeform Fabrication of Stainless Steel Using Fab@Home", Proceedings of the 19th Annual Solid Freeform Fabrication Symposium, Austin TX, Aug 2008. PDF
- Malone, E. (2006). Faxing Artifacts: The promise of three-dimensional scanning and rapid prototyping of archaeological materials, International Council of Archaeo-Zoologists – ICAZ 2006, Mexico City, Mexico, 23-28 Aug 2006, Poster.
- Malone E., Lipson H., (2006) "Freeform Fabrication of Ionomeric Polymer-Metal Composite Actuators", Rapid Prototyping Journal, Vol. 12, No. 5, pp.244-253.
- Malone E., Lipson H., (2007) "Fab@Home: The Personal Desktop Fabricator Kit", Rapid Prototyping Journal, Vol. 13, No. 4, pp.245-255. PDF. (This is to our knowledge the best easy to read article explaining most aspects of a desktop fabricator).
- Malone E., Rasa K., Cohen D. L., Isaacson T., Lashley H., Lipson H., (2004) "Freeform fabrication of 3D zinc-air batteries and functional electro-mechanical assemblies", Rapid Prototyping Journal, Vol. 10, No. 1, pp. 58-69.
- Malone, E., Berry, M., Lipson, H., (2008), "Freeform Fabrication and Characterization of Zinc-air Batteries", Rapid Prototyping Journal, Vol. 14, No. 3, pp. 128-140. PDF
- Martin, Lee (2015) "The Promise of the Maker Movement for Education," Journal of Pre-College Engineering Education Research (J-PEER): Vol. 5: Iss. 1, Article 4. http://dx.doi.org/10.7771/2157-9288.1099
- Apoorva Mandavilli (2006). Appropriate technology: Make anything, anywhere, Nature 442, 862-864 (24 August 2006). doi:10.1038/442862a.
- McMains, S. (2005). "Layered Manufacturing Technologies", Comm. ACM, Volume 48, Number 6, pp 50-56.
- Mikhak, Bakhtiar; Christopher Lyon, Tim Gorton, Neil Gershenfeld, Caroline McEnnis and Jason Taylor (unknown). Fab Lab: An Alternate Model Of Ict For Development, PDF
- Milanese, Fiona (2006). FABLab allows you to create the gadget of your dreams, Science in Africa. August 2006, HTML
- Montaro, M. et al., 2002, “Material Characterization of Fused Deposition Modeling (FDM) ABS by Designed Experiments,” University of California, Berkeley Report.
- Moon, F. C., 2003a, Franz Reuleaux: Contributions to 19th Century Kinematics and Theory of Machines, Applied Mechanics Reviews, American Society of Mechanical Engineers, N.Y.
- Oblinger, D.G. and J.L. Oblinger (2005). Educating the net generation, in Chapter 2: Is it age or IT: First steps toward understanding the net generation, D.G. Oblinger and J.L. Oblinger, Editors. 2005, Educause: Washington, D.C. p. 8.
- Qualifications and Curriculum Authority (QCA). (1999). The national curriculum for England: Design and technology. London: DfEE/QCA.
- Sells, Ed; Zach Smith, Sebastien Bailard, and Adrian Bowyer (2007). RepRap: The Replicating Rapid Prototyper - Maximizing Customizability by Breeding the Means of Production. Extended abstract in Proc. Mass Customization and Personalization Conference, MIT, October 2007.
- Spendlove, David (2007). The locating of emotion within a creative, learning and product orientated design and technology experience: person, process, product. International Journal of Technology and Design Education, Volume 18, Number 1, 45-57, DOI 10.1007/s10798-006-9012-2
- Steeg, T., 2008. Makers, Hackers and Fabbers: what is the future for D&T? IN: Norman, E.W.L. and Spendlove, D. (eds.). The Design and Technology Association International Research Conference, [Loughborough University, 2-4 July]. Wellesbourne : The Design and Technology Association, pp. 65-73. 08.pdf PDF - HTML Abstract. (includes throughts about Design and Technology teaching)
- Thomson, Clive (2008). Build It. Share It. Profit. Can Open Source Hardware Work? Wired Magazine, 16:1
- Touchgraphics, Inc. (2009). An Interactive Talking Campus Model at Carroll Center for the Blind, Final Report, center talking campus model final report low.pdf PDF. (Objects were created with a commercial 3D printer).
- Vilbrandt, T., Malone, E., Lipson H., Pasko, A., (2008) "Universal Desktop Fabrication", in Heterogeneous Objects Modeling and Applications, pp. 259-284. DOI: 10.1007/978-3-540-68443-5 (Access restricted)
- Walter-Herrmann J, Büching C, editors. FabLab: Of machines, makers and inventors. transcript Verlag; 2014.
- Wohlers, T. (2005). 3D Printing in Education: How High Schools, Colleges, and Universities Leverage 3D Printing Technology.â Time-Compression Technologies, Sept/Oct 2005. HTML