Fab labs in education
This article or section is currently under construction
In principle, someone is working on it and there should be a better version in a not so distant future.
If you want to modify this page, please discuss it with the person working on it (see the "history")
<pageby nominor="false" comments="false"/>
Introduction
See also:
- Fab lab (Technical and political overview)
- Tour de Fablab (Some ressources for demos/workshops)
- 3D printers in education
- Computerized embroidery in education
- Digital design and fabrication in education
Fab labs in education seem to follow several connected axis of development. For example:
- Teaching of "design" through modelling and physical realisation
- Creation of manipulables (e.g. bricks of a warehouse) or persona
- Models for teaching about past or present articifacts or abstract concepts
- Having learners create such objects themselves.
Conceptual foundations can be rooted in constructionism or even older theories, but the relative affordance of fabbing tools in the early 2000's gave birth to a round of new Research and Development (e.g. Andersen et al, 2005 or Gershenfeld, 2005). In 2009, prices for devices like a 3-D printer a low enough for school budgets or even individuals.
As the power of desktop fabbers will increase and cost decrease over the next few years, Lipson et al. (2004:1032) argue that “more elaborate machines may be printable by a growing community around the world. Moreover, as new research leads to multimaterial functional freeform fabrication, we expect that incorporation of elastomers, lubricants, actuators, and sensors, electronics and power devices (Lipson, 2005b) will allow faithful replication and electronic sharing of an ever increasing scope of physical models and artifacts.”
Teaching of design and fabrication
Fab labs were born in higher education (e.g. Gershenfeld:2005) and most of these are sponsored by academic institutions. Of course, technical hobbyists always did exist and and in many countries, schools do offer facilities and even classes for all sorts of bricolage. The most prominent fabber projects were founded by academics. E.g. RepRap by Adrien Bowyer et al. at University of Bath (UK); Fab@Home by Evan Malone while he was a PhD student at Cornell. Commercial low-cost printers are sold as tool for design classes. E.g. a Desktop Factory printer is advertised as “With the Desktop Factory 3D printer, departments within large firms will be able to have their own dedicated 3D printers, and many small businesses, design firms and schools will be able to own this capability for the first time. Professional designers, engineers and students alike will be able to build inexpensive models from their designs before committing to expensive, custom prototyping” (retrieved 17:30, 25 June 2009 (UTC)).
While we will not discuss in detail obvious application areas of "fab lab techniques" in research and design, there exist a number of areas where cheap "fab lab" technology and in particular 3-D printers could be successfully used. E.g. Allard (2006) argues that “3D printing has the potential to overcome the barriers to the widespread use of RP in biological anthropology. The 3DP technology is easy to use, fast and economical to operate making it well suited to supporting projects in biological anthropology. The main limitation of the use of 3DP is the level of awareness of how it can be used to enhance or facilitate projects in biological anthropology.”
Burry (2006) analysis Antoni Gaudi's approach to thinking, modeling, and making. “[...] in considering the model as a design instrument, and modelling as a design process, Gaudi has bequeathed us two bases for reflecting on how we make and use models today. The first is his creation of a unique process based on a geometrical codex whose value is shared by designer and builder alike. The second bequest is a process involving an apprenticeship that has stood the test of time inasmuch as the exact methods that he developed in his time are still in abundant use on the project today.”
These two examples illustrate that there is interest for "rapid prototyping" techniques in diverse areas outside of engineering, and that maybe should be taught in school, i.e. 3D scanning, modelling and 3D printing may enter curricula.
Models for education
Knapp et al. (2007) stress the benefits of physical models in a variety of educational settings, e.g. Mathematics, anatomy, molecular biology, aeronautics, chemistry, archeology: “Physical models have been shown to enhance learning in general student populations as well. Students learn in a variety of ways, and models allow students to include their sense of touch in the learning experience. The role of experience is emphasized in Piaget's description of cognitive development, that is, to know an object a subject must act on it and thus transform it - displace, connect, combine, take apart, and reassemble it. (Cohen, 1983).
Historic artifacts
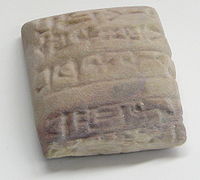
Lipson et al. (2004:1032) reports on “the use of computer-aided modeling tools and rapid prototyping technology to document, preserve, and reproduce in three dimensions, historic machines, and mechanisms. We have reproduced several preassembled, fully functional historic mechanisms such as early straight line mechanisms, ratchets, pumps, clock escapements and counting devices, including various kinematic components such as links, joints, gears, worms, nuts, bolts, and springs.”. The authors also argue that “One can realize many historical concepts that exist only on paper, such as Leonardo da Vinci's slider crank mechanism, as well as other models that exhibit more contemporary concepts from aerodynamics to molecular biology.”
Physical models of abstract concepts
Science education has a long tradition of using of models. If one goal of science education is to enhance and maximize an individual's special conceptual ability, then access to manipulatives is advisable for those individuals. This access to manipulatives might also enhance development of their logical abilities... Internality is positively correlated with student achievement, and experience with manipulatives tends to move external subjects toward the internal end of the internal external continuum. (Cohen, 1982)”. Knapp et al. also stress the general benefits of models and manipulatives, quoting a study from Lillard (2006) showing that children from a Montessori kindergarten significantly outperformed their peers at traditional schools in standardized tests of reading and math.
In mathematics education, for example, Eisenberg et al. already in 2005 made the following statement. “One of the most important shifts in mathematical crafts is due to the increased power and affordability of fabrication devices - essentially, new sorts of output devices - that work in conjunction with computers. (Cf. the recently-published book by Gershenfeld for an enthusiastic discussion.) There are a number of devices that are relevant to this theme, among which are: (i) laser cutters (which employ a laser to cut flat sheets of wood or plastic into desired shapes), (ii) 3D printers (which output 3-dimensional forms in plastic or plaster, among other possible materials), and (iii) computer-controlled sewing machines (which can embroider fabric according to computational control)”.
Among other benefits these authors point that “One usually-unheralded aspect of mathematical crafts (particularly in comparison to purely "virtual", computer-based activities) is that the use of tangible materials permits children to create objects and artifacts that populate their physical space. In classrooms, it is not all that unusual to see mathematical models placed on shelves, hung from the ceiling, assembled into mobiles, and so forth. The contrast with computer-based activities here is telling: a mathematical game or simulation may be marvelous, but it remains (for the most part) "hidden" inside the computer; unlike the physical products of crafts, which are simply present and continuous in children's spaces, an educational computer program is invisible unless consciously accessed.”
Building blocks for games and simulations
Computer supported physical manipulatives
A good example is the TinkerTable integrated learning environment. It is a “tabletop learning environment which allows apprentices to build small-scale models of a warehouse using physical objects like wooden shelves, docks and rooms as well as metallic pillars, all scaled at 1:16. The system is made of a 2m by 1.5m table covered with whiteboard material and a gallows carrying a camera, a projector and a mirror. The purpose of the camera is to track the position of objects on the table and transfer this information to a computer running a logistics simulation. The position of the object is obtained thanks to fiducial markers (similar to 2D barcodes) detected by StudierStube tracker. The projector is used to project information on the table and on top of the objects, indicating for example the accessibility of the content of each shelf or security zones around obstacles.” ( retrieved 13:44, 25 October 2009 (UTC))
“The TinkerLamp environment is a lighter and portable version of the TinkerTable. It consists of a projector and camera mounted in a metal casing which is suspended above a regular classroom table by an aluminum gooseneck. Shelves, pillars and docks are scaled at 1:48. The functionality of this small version is identical to the large version except for the size of the warehouse (32m by 24m on the TinkerTable but limited to 24m by 18m) on the TinkerLamp version”. The shelves (i.e. the manipulatives) were made with ABS plastic and could have been printed with a 3D printer.
Objects for the visually impaired
The Tactilelearning.org stresses the importance of tactile models for the visually impaired and present several devices made "by hand" for teaching. A similar project is Touch Graphics that produced a number of projects, some of which are commercially available.
Eisenberg et al (2005) demonstrated for example embedded computers within construction pieces that are used to create two dimensional and three-dimensional "cellular automaton kits". “By placing computers inside craft objects themselves, we can make those objects programmable, and can endow them with qualities of interactivity and autonomous behavior. In turn, this enables new sorts of mathematical content to be brought into the sphere of craft activities.”
Social issues and development
Sharing
Several repositories now exist that allow people to share (and take). Most 3D printers rely on the STL format described above and that decomposes a 3D structure into slices. There exist several options to create such a printable 3D representation.
Since most (active) teachers do want to adapt designs to their own needs, sharing of the model is not enough. Source code also must be shared, and ideally designers in various sub-fields also should share the same tools and ways of producting artifacts. For example, models for math teaching can be created with the following workflow desribed by Knapp et al. (2008):
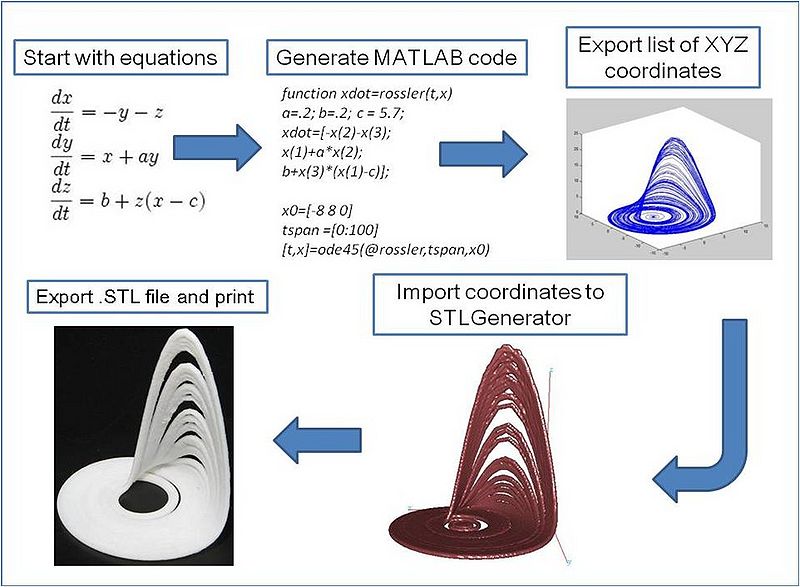
Others may use 3D modeling software or 3D scanners.
Bibliography
- Allard, Travis, T. (2006). The Role of 3D Printing in Biological Anthropology, University of Manitoba, Master Thesis, Allard 2006.pdf PDF
- Andersen, D; C. Bennett, P. Huynh, L. Rassbach, S. Reardon, and M. Eisenberg (2005). Printing Out Trees: Toward the Design of Tangible Objects for Education, Proceedings of Education and Technology. PDF.
- Bereza, Marek (2007). Rise of the Replicator: The Evolution of Media Into The Tangible. The Royal College of Art, Master thesis. PDF
- Breen, Jack; Robert Nottrot, Martijn Stellingwerff (2003). Tangible virtuality--perceptions of computer-aided and physical modelling, Automation in Construction, Volume 12, Issue 6, Design e-ducation: Connecting the Real and the Virtual, November 2003, Pages 649-653, ISSN 0926-5805, DOI: 10.1016/S0926-5805(03)00053-0.
- Burns M., (1995) The Freedom to Create, in Technology Management, Volume 1, Number 4 http://www.ennex.com/~fabbers/publish/199407-MB-FreedomCreate.asp
- Bowyer, Adrian (2007) Why Accountants are Dull and Guitarists are Glamourous - The End of Intellectual Property, Time Compression Technology Magazine, volume 15, issue 3, p33 (2007).
- Bowyer, Adrian (2007)- The Self-replicating Rapid Prototyper ─ Manufacturing for the Masses, Invited Keynote Address, Proc. 8th National Conference on Rapid Design, Prototyping & Manufacturing, Centre for Rapid Design and Manufacture, High Wycombe, June 2007. Rapid Prototyping and Manufacturing Association, ISBN-13: 978-0948314537 (2007).
- Bowyer, Adrian (2007). Breed your own Design, Icon Magazine, volume 52, October 2007.
- Burry, Mark; Michael Ostwald, Peter Downton, Andrea Mina, (2007) Homo Faber - Modelling Architecture, Sydeney: Archadia Press, ISBN 9780977571123. PDF (See also the video: Thinking modeling making
- Ceccarelli, M., (Ed.) 2000, International Symposium on History of Machines and Mechanisms, Proc. HMM Kluwer Academic, Dordrecht.
- Cohen D. L., Malone E., Lipson H., Bonassar L., (2006) "3D direct printing of heterogeneous tissue implants", Tissue Engineering, Vol. 12, No. 5: 1325-1335
- Cohen, H.G. (1983). A comparison of the affect of two types of student behavior with manipulatives on the development of projective spatial structures. Journal of Research in Science Teaching, 20(9), 875-883
- Cohen, H.G. (1982). Relationship between locus of control and the development of spatial conceptual abilities. Science Education, 66(4), 635-642
- Dlodlo, Nomusa and Ronald Noel Beyers (2009). The Experiences of South-African High-School Girls in a Fab Lab Environment, Proceedings Of World Academy Of Science, Engineering And Technology Volume 37 January 2009 Issn 2070-3740. PDF Reprint
- Editors' Review (2005). Desktop Factories - FAB The Coming Revolution on Your Desktop -- from Personal Computers to Personal Fabrication By Neil Gershenfeld, Basic Books, Business Week, May 2 2005.
- Eisenberg, Michael, Ann Eisenberg, Glenn Blauvelt, Susan Hendrix, Leah Buechley, and Nwanua Elumeze (2005). Mathematical Crafts for Children: Beyond Scissors and Glue, Proceedings of Art+Math=X Conference, Boulder, CO, pp. 61-65, 2005. Reprint: Department of Computer Science, University of Colorado. PDF
- Eisenberg, M. and Buechley, L. (2008). Pervasive Fabrication: Making Construction Ubiquitous in Education Journal of Software, 3:4, pp. 62-68. PDF
- Evil Mad Scientist Laboratories (2007). Solid freeform fabrication: DIY, on the cheap, and made of pure sugar, http://www.evilmadscientist.com/article.php/candyfab, retrieved 17:30, 25 June 2009 (UTC).
- Gershenfeld N. Think Globally, fabricate locally, PrincipalVoices.com. PDF (reprint)
- Gershenfeld, Neil, A., (2005) FAB: The Coming Revolution on Your Desktop – From Personal Computers to Personal Fabrication, Basic Books, ISBN 0-465-02745-8.
- Institute of the Future (2009). The future of making, PDF
- Kuznetsov, Stacey and Eric Paulos (2010). Rise of the Expert Amateur: DIY Projects, Communities, and Cultures, Proceedings of ACM NordiCHI 2010: Extending boundaris, Reykjavik, PDF preprint
- Jenweill, Mark, Fab Labs unshackle imaginations, USA Today, 11/6/2005.
- Jensen, Daniel; Chris Randell, John Feland, Martin Bowe (2002). A Study Of Rapid Prototyping For Use In Undergraduate Design Education, Proceedings of the 2002 American Society for Engineering Education Annual Conference & Exposition. PDF
- Moon, F. C., 2003a, Franz Reuleaux: Contributions to 19th Century Kinematics and Theory of Machines, Applied Mechanics Reviews, American Society of Mechanical Engineers, N.Y.
- Lillard, A. and Else-Quest, N. (2006) The Early Years: Evaluating Montessori Education Science, Vol. 313. no. 5795, pp. 1893 - 1894
- Lipson H., Moon F.C., Hai J., Paventi C., (2004) 3D-Printing the History of Mechanisms, ASME Journal of Mechanical Design, pp. 1029-1033. PDF
- Lipson H. (2005a) "Homemade: The future of Functional Rapid Prototyping", IEEE Spectrum, feature article, May 2005, pp. 24-31 http://www.mae.cornell.edu/ccsl/papers/Spectrum05_Lipson.pdf
- Lipson H. (2005b). "Homemade: The future of Functional Rapid Prototyping", IEEE Spectrum, feature article, May 2005, pp. 24-31.
- Lipson (2007), Printed 3D Models for Customized Hands-On Education. Proceedings of Mass Customization and Personalization (MCPC) 2007.
- Knapp M., Wolff R., Lipson H. (2008), "Developing printable content: A repository for printable teaching models", Proceedings of the 19th Annual Solid Freeform Fabrication Symposium, Austin TX, Aug 2008. PDF.
- Lobovsky M., Lobovsky A., Behi M., Lipson H. (2008), "Solid Freeform Fabrication of Stainless Steel Using Fab@Home", Proceedings of the 19th Annual Solid Freeform Fabrication Symposium, Austin TX, Aug 2008. PDF
- Malone, E. (2006). Faxing Artifacts: The promise of three-dimensional scanning and rapid prototyping of archaeological materials, International Council of Archaeo-Zoologists – ICAZ 2006, Mexico City, Mexico, 23-28 Aug 2006, Poster.
- Malone E., Lipson H., (2006) "Freeform Fabrication of Ionomeric Polymer-Metal Composite Actuators", Rapid Prototyping Journal, Vol. 12, No. 5, pp.244-253.
- Malone E., Lipson H., (2007) "Fab@Home: The Personal Desktop Fabricator Kit", Rapid Prototyping Journal, Vol. 13, No. 4, pp.245-255. PDF. (This is to our knowledge the best easy to read article explaining most aspects of a desktop fabricator).
- Malone E., Rasa K., Cohen D. L., Isaacson T., Lashley H., Lipson H., (2004) "Freeform fabrication of 3D zinc-air batteries and functional electro-mechanical assemblies", Rapid Prototyping Journal, Vol. 10, No. 1, pp. 58-69.
- Malone, E., Berry, M., Lipson, H., (2008), "Freeform Fabrication and Characterization of Zinc-air Batteries", Rapid Prototyping Journal, Vol. 14, No. 3, pp. 128-140. PDF
- Apoorva Mandavilli (2006). Appropriate technology: Make anything, anywhere, Nature 442, 862-864 (24 August 2006). doi:10.1038/442862a.
- McMains, S. (2005). "Layered Manufacturing Technologies", Comm. ACM, Volume 48, Number 6, pp 50-56.
- Mikhak, Bakhtiar; Christopher Lyon, Tim Gorton, Neil Gershenfeld, Caroline McEnnis and Jason Taylor (unknown). Fab Lab: An Alternate Model Of Ict For Development, PDF
- Milanese, Fiona (2006). FABLab allows you to create the gadget of your dreams, Science in Africa. August 2006, HTML
- Montaro, M. et al., 2002, “Material Characterization of Fused Deposition Modeling (FDM) ABS by Designed Experiments,” University of California, Berkeley Report.
- Sells, Ed; Zach Smith, Sebastien Bailard, and Adrian Bowyer (2007). RepRap: The Replicating Rapid Prototyper - Maximizing Customizability by Breeding the Means of Production. Extended abstract in Proc. Mass Customization and Personalization Conference, MIT, October 2007.
- STEEG, T., 2008. Makers, Hackers and Fabbers: what is the future for D&T? IN: Norman, E.W.L. and Spendlove, D. (eds.). The Design and Technology Association International Research Conference, [Loughborough University, 2-4 July]. Wellesbourne : The Design and Technology Association, pp. 65-73. 08.pdf PDF - HTML Abstract. (includes throughts about Design and Technology teaching)
- Thomson, Clive (2008). Build It. Share It. Profit. Can Open Source Hardware Work? Wired Magazine, 16:1
- Touchgraphics, Inc. (2009). An Interactive Talking Campus Model at Carroll Center for the Blind, Final Report, center talking campus model final report low.pdf PDF. (Objects were created with a commercial 3D printer).
- Vilbrandt, T., Malone, E., Lipson H., Pasko, A., (2008) "Universal Desktop Fabrication", in Heterogeneous Objects Modeling and Applications, pp. 259-284. DOI: 10.1007/978-3-540-68443-5 (Access restricted)
- Wohlers, T. (2005). 3D Printing in Education: How High Schools, Colleges, and Universities Leverage 3D Printing Technology.â Time-Compression Technologies, Sept/Oct 2005. HTML